Solar 101
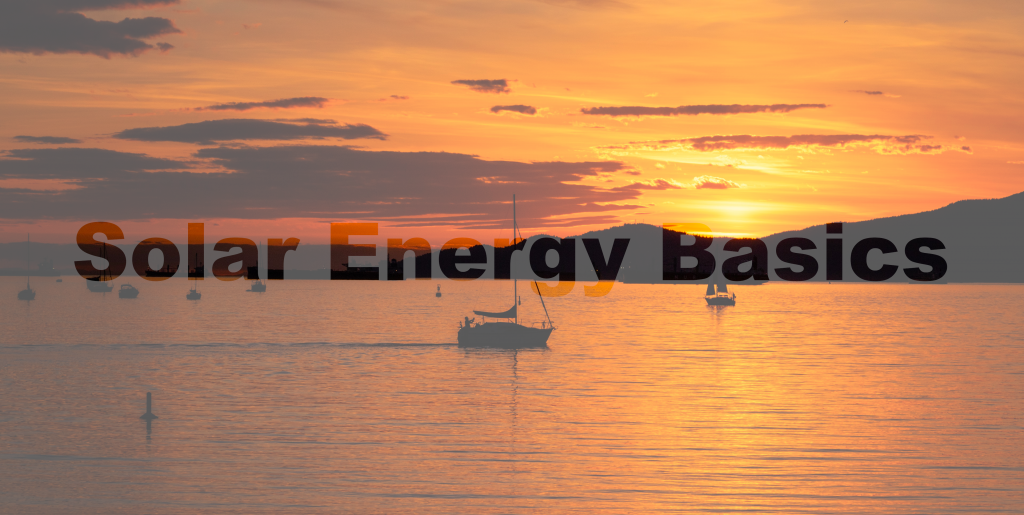
Solar Photovoltaic Energy Basics
Solar energy is a sustainable source of energy that captures the sun’s radiation and transforms it into useable electricity that can power our communities. Photovoltaic (PV) cells capture solar energy; inverters convert the solar power into usable electricity. Specifically, it converts the direct current (DC) power collected from the PV cells to alternating current (AC). This is essential because our electrical appliances use AC power. Solar panels are collections of PV cells packaged together to form a useful source of power. Connecting many of these solar panels will form an array that is substantial enough to power a single boat, a large house, or even an entire community.
Some communities use solar power to supplement the power their local power companies provide. Other communities generate all their electrical power with solar panels or other renewable energy sources and operate independently of the power grid. In other words, there are two types of usage for these PV systems:
- Grid-tied, which work cooperatively with the local grid by feeding it electricity generated from the panels; or
- Off-grid, which operate independently of the power grid and store electricity in batteries.
Depending on your needs for the system, additional parts may be necessary. For all off-grid purposes, a charge controller will be needed to charge the battery that the electricity is being stored in. This is also true for grid-tied systems that have a backup battery bank. Batteries for grid-tied systems are not necessary, but are ideal for emergency use when the grid power unexpectedly fails.
Measuring Solar Energy as Electricity
There are quite a number of different measures and units when it comes to electricity that you will need to know for your solar PV system. Let’s try to break them down in the simplest terms.
Voltage (V) refers to the energy potential of a system, usually described as the intensity of the current or how fast it is moving. Voltage is also useful in determining the compatibility of electrical appliances. Say for example you have a 24V inverter and you wish to charge a battery for a boat; the only batteries that will work for this inverter must match the 24V. One can do this by either using a 24V battery, or by attaching two 12V batteries in parallel, which will effectively produce a 24V battery that will be compatible with the 24V inverter.
The Amperage (A) is the amount of electrical current that can flow through a system. Generally speaking, the thicker a wire is, the more amps it can carry. Some appliances require more electricity to flow through them, so a larger wire with a higher amp load would be necessary. Heat energy will be transmitted if the amp load of a wire does not transfer enough electricity for an appliance, which could lead to a fire.
V x A = W
The capacity of power these cells and panels generate is measured in watts (W), which is the amount of energy it can produce per second. More commonly, thousands of watts are expressed in kilowatts (kW) for larger figures of this measure. The amount of energy the system generates is expressed in kilowatt hours (kWh). A kWh is equal to the amount of kilowatts produced or consumed per hour. The amount of kilowatt hours a system generates is determined by the capacity of the system, as well as the intensity and duration of sunlight radiation received by a panel. We will discuss this in more detail below.
Locating Panel Arrays
Finding the best place to rest one’s panels is crucial. Depending on the size of your system, you may be constrained by the amount of area you have available. This is why it is imperative to measure out your desired area to ensure your system has enough space. Most commonly, fixed panels are placed on rooftops with stable racks. This calculation is much simpler since the panels can lay side by side on the surface and a rectangular shape (use length x width calculation).
When we introduce alternate panel mounts that raise the solar panels above the surface, like our sun tracking dynamic panel systems, the area calculation becomes much more complex. This is because when panels are raised above a surface, they will create a shadow which may interfere with other nearby panels.
Efficiency and Solar Angles
The power generated by solar PV panels depends on the intensity of incoming radiation from the sun. The intensity of the incoming radiation largely depends on the angle of incidence – that is, the angle between the surface normal of the panel’s tilt and the sun’s rays. The surface normal is a perpendicular line or ray from the surface of the solar panel. Essentially, the smaller the incidence angle of the panel and the sun’s radiation, the greater the efficiency output your solar panel will achieve. This angle is calculated in three-dimensions, but we will demonstrate it in two-dimensions for convenience.

We must also take into account the horizontal directional plane when determining the optimal angle for a solar panel. This will add a third dimension to our equation of this angle, which is not shown in the above diagram. To do this, we must think of which direction our panel should be facing. For those in the northern hemisphere, the sun will always appear in the southern sky, moving east to west. The angle between the horizontal position of the sun in and directly south is what’s known as the solar azimuth angle. This angle is crucial in determining the optimal horizontal direction for a solar panel to face. The horizontal direction the solar panel is facing with respect to the southern horizontal is the surface azimuth angle. The closer both the solar and surface azimuth angles are together, the greater the efficiency the solar panel will realize from the horizontal dimension.
Putting all of these dimensions together will determine the useful angle of incidence. That is, once two angles of a triangle are found, the third angle can be determined. For our purposes, the vertical and horizontal angles of incidences yields the three-dimensional angle of incidence. We will use this to optimize the solar panel’s tilt and direction which will maximize our panel system’s efficiency.
The efficiency of a panel describes the amount of energy being produced relative to its capacity for production, expressed in a percentage. A solar panel in perfect working condition on a clear and sunny day with zero angle of incidence will produce at 100% efficiency. At night, a solar panel will not be generating any energy, so it will be working at 0% efficiency. If the goal is to maximize the solar panels’ efficiency for as long as possible, then a fixed panel system is not ideal. Luckily, we have a solution to this.
Solar Tracking and Sensors
Since the sun’s position in the sky changes drastically throughout the day and year, the angle of incidence will also be subject to constant change. Consequently, normal fixed panels will lose a great amount of efficiency even if they are fixed at the most optimal angles. This is where the dynamic solar panels with sun-tracking technology are massively beneficial. This new technology is the solution to these efficiency losses, since it allows a solar panel to adjust its surface normal so that it will maintain a very low angle of incidence. Remember, the lower the angle of incidence, the greater your panel’s efficiency output will be.
Our innovative Sunflower technology addresses these efficiency losses because it is designed to constantly minimize the level of incidence between the sun and the panel.
There are many different methods of sun-tracking which all have their own benefits and drawbacks. However, two main categories of accurate tracking are available:
- Open-loop: uses location and time specific data inputs to output the optimal panel orientation.
- Closed-loop: uses at least two optical sensors and a microprocessor to determine the location of the sun in the sky.
Since we can easily find out the optimal angles to position a solar panel at any given location and time, we can program these formulas into a computer which can then tell the panel to rotate or tilt to output the desired angle of incidence. The main problem with these open-loop trackers is that they require energy to compute the optimal panel orientation. This means there needs to be a significant supply of energy to run the tracker. Energy used to power this tracking system will offset the net energy gains from the whole system, which may drastically reduce the overall net energy produced.
Optical sensors for closed-loop systems can vary significantly in their design and performance. To put briefly, these sensors detect where the sun is in the sky relative to the panel location, which is then communicated to the panel via electronics and software code. The main drawback to these sensors is that there can be interference with the sunlight detection due to environmental factors, such as clouds and dust.
Investing in Solar PV Energy
Many people see a solar PV system as an expensive alternative to traditional energies. In reality, it is an investment with a long-term focus. When you factor in the amount of energy the system produces, the cost of energy in your area, and the upfront costs of installing a system, most systems will pay for themselves within 4-10 years. It should be noted that any financial benefits received after the payback period is fulfilled is effectively pure savings. There are many financial incentives in place to reduce the upfront costs and shorten the payback period of a system, especially in Canada.
To calculate the payback period for a solar panel system, we will need to know three things:
- The total system installation and upfront costs (subtract any tax breaks, rebates, or any other external funding)
- The regional rate for energy consumed or produced in kWh
- The total amount of kWh the system will produce annually